Mechanochemistry
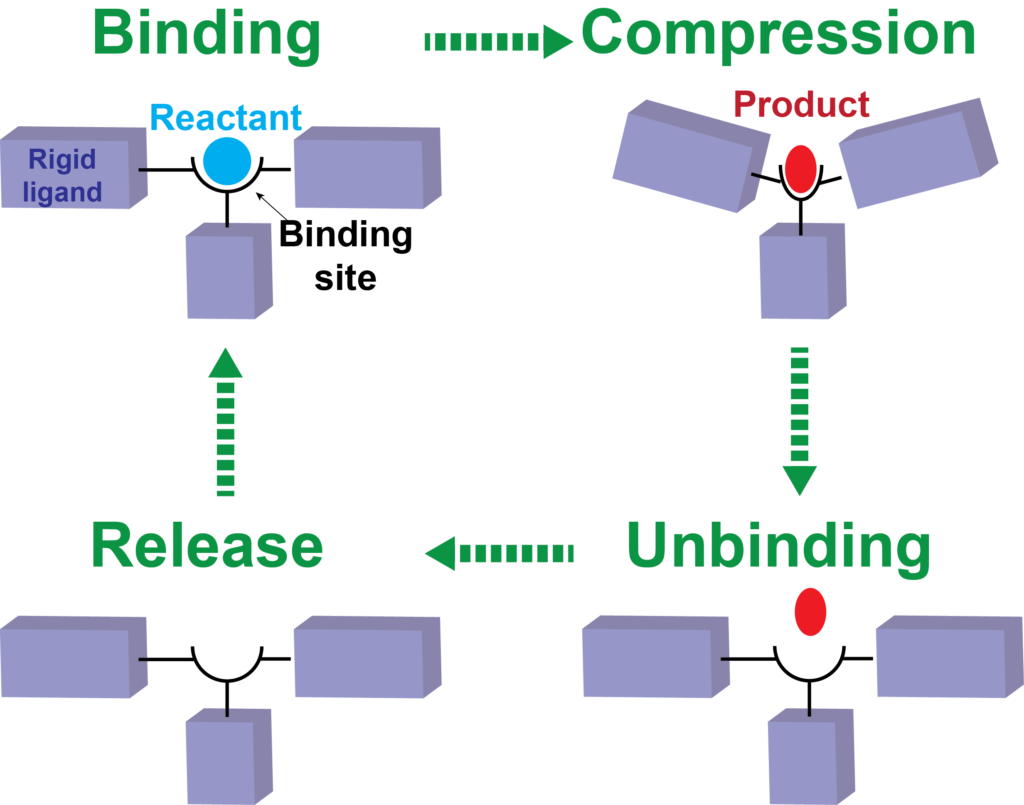
We are inspired by the notion that mechanical stress can alter molecular structures, conformations and reaction energy landscapes. This presents some very interesting opportunities to re-examine fundamental concepts such as the structure-property relationship beyond the existing realms of chemistry.
We strive to answer the following questions: how are macroscopic forces translated into molecular-scale deformations? How are these deformations correlated to their chemical properties? The answers to these questions will lead to new design principles of molecular machinery that carries out chemical transformations under mechanical drive. For example, one can conceive a ‘reversed combustion engine’ – molecules that utilize mechanical energies to make fuels and commodity chemicals. These ideas will fundamentally change how we think about synthesis, catalysis and energy conversion.
Chemical functionality in extreme environments
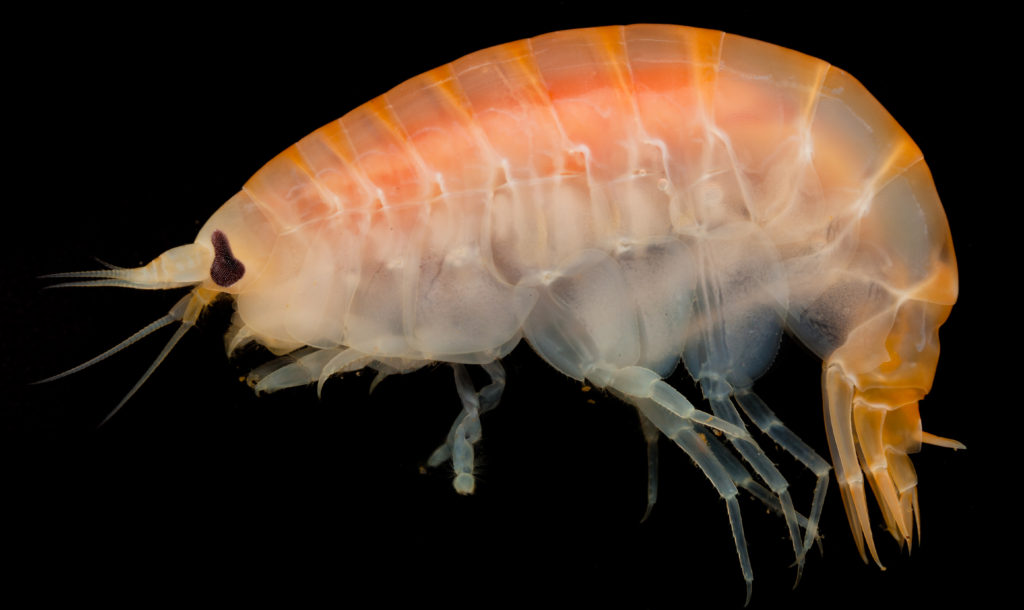
Roughly 70% of the global ecosystem exists in non-ambient environments. Species like the H. gigas amphipods thrive under hundreds of megapascal pressure, which would be fatal for ‘normal’ forms of life. These organisms, known as piezophiles, developed unique mechanisms to cope with the detrimental effects of high pressure including protein denaturation and cell membrane stiffening. The molecular foundations of these mechanisms, however, are yet to be fully understood.
Mechanochemistry provides a unique angle toward these questions. Using model systems, we investigate how basic chemical processes, from electron transport to catalysis, are affected by high pressure, and how these effects are modulated by the mechanical properties of molecules and ensembles. Through these studies we hope to gain crucial insight into the early stage of life on Earth, and draw inspirations for new functional materials that endure harsh conditions.
Metastable materials
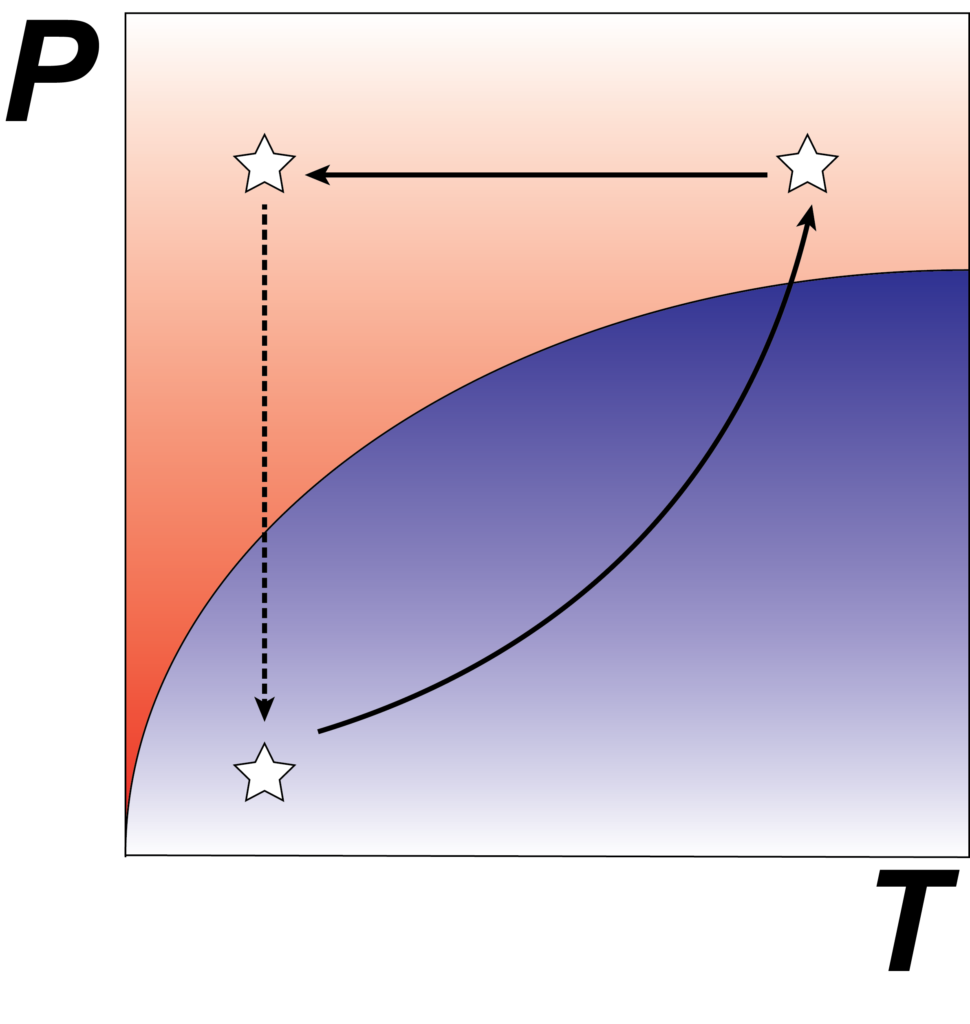
A diamond may be forever, yet from the thermodynamic perspective it is less stable than graphite at ambient pressure. Nature utilizes high pressure to favor the formation of the diamond phase, which is kinetically trapped when pressure is removed. We adopt the same principle for the discovery of metastable materials that are inaccessible via conventional synthesis, with particular emphasis on establishing fine-grain structural controls such as dimensionality and atomic defects.